Summary: A new paper offers an overview as to how neurons ‘communicate’ with one another.
Source: Max Planck Institute.
Neurons are connected to each other through synapses, sites where signals are transmitted in the form of chemical messengers. Reinhard Jahn, Director at the Max Planck Institute for Biophysical Chemistry in Göttingen, has investigated precisely how the process works.
Our nervous system consists of about 100 billion interlinked neurons that are capable of carrying out complex computations. Each neuron has an antenna zone comprising the cell body and its extensions (dendrites). It is here that it receives signals from other neurons.
One cell talks, the other listens
The signals are then computed and forwarded by a “cable”, the axon, in the form of electrical impulses. In the emitter region, the axon branches to form contact sites, known as synapses, where the signals are transmitted to other neurons. At the synapse, electrical impulses arriving from the axon are converted into chemical signals. The information then flows in only one direction: one cell talks, the other listens. The number of synapses that a single neuron can develop varies considerably. Depending on its type, a neuron can have anything from just one to more than 100,000 synapses. On average, each neuron has around 1,000 synapses.
Synapses – elementary units of neuronal information transmission
Synapses consist of:
- the nerve ending of the transmitting (presynaptic) neuron,
- the synaptic cleft separating the transmitting and receiving neurons and
- the membrane of the receiving (postsynaptic) neuron.
The presynaptic nerve endings contain signal molecules known as neurotransmitters, which are stored in small membrane-enclosed vesicles. Each nerve ending in the central nervous system contains an average of several hundred synaptic vesicles. However, synapses vary significantly. For example, some specialist synapses contain more than 100,000 vesicles. They include the synapses that control our muscles. At each synapse some vesicles are always in the starting position, “lurking”, as it were, on the presynaptic plasma membrane to which they have docked.
Molecular machines at work
An electrical signal arriving at the nerve ending activates calcium channels in the plasma membrane. Calcium ions from outside then flow through the channels into the interior of the synapse. There, the inflowing calcium ions encounter and activate a molecular machine located between the vesicle membrane and the plasma membrane. This machine causes the membranes of the vesicles in the starting position to fuse with the plasma membrane and release the neurotransmitters contained in the vesicles into the synaptic cleft.
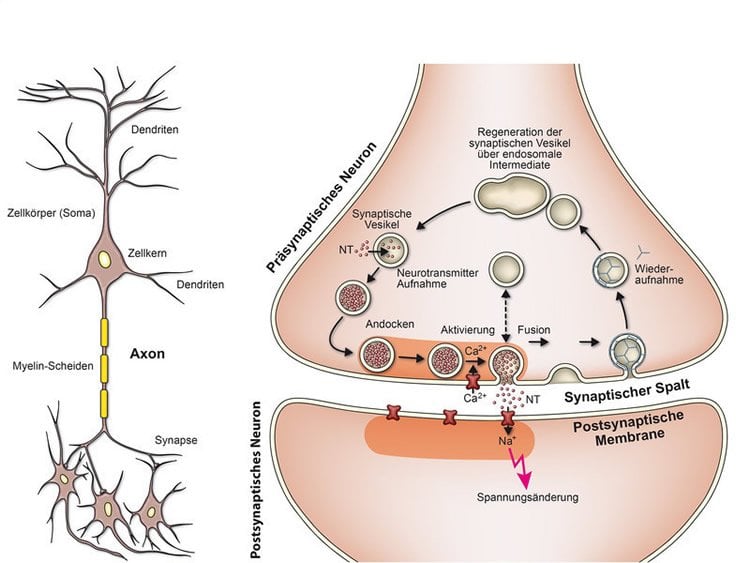
On the other side of the synaptic cleft, the neurotransmitters come into contact with docking sites on the membrane of the receiving neuron which regulate the electrical properties of that membrane. This alters the electrical resistance of the membrane. The receiving cell is able to process the resulting potential change rapidly. Only around one thousandth of a second elapses between the arrival of an impulse and the potential change on the other side of the synaptic cleft. In fact, synaptic transmission is one of the fastest biological processes known. Nevertheless, it is positively sluggish in comparison to a transistor.
Synaptic vesicles: not just storage organelles
A synaptic vesicle is not just a kind of membrane-bound “storage tank” for neurotransmitters. Its membrane contains a whole series of proteins that have barely changed over millions of years of evolution. A group of these proteins, the neurotransmitter transporters, are responsible for pumping neurotransmitters from the cytoplasm into the vesicles, where they accumulate. This process requires a great deal of energy, which is provided by another protein molecule, proton ATPase (V-ATPase), that pumps protons into the vesicles – a process that is fuelled by adenosine triphosphate (ATP). The pumps, in turn, use the resulting concentration gradient for the uptake of neurotransmitters.
In addition to these proteins required for “replenishing”, the membranes of synaptic vesicles contain other components that enable the vesicles to fuse with the plasma membrane (including the SNARE protein synaptobrevin and the calcium sensor synaptotagmin). Once membrane fusion has occurred, they are transported back into the nerve ending. The synaptic vesicles are then recycled back into the nerve ending via several intermediate steps and are refilled with neurotransmitters. This process is repeated again and again, thousands of times in the life cycle of a vesicle.
The process by which synaptic vesicles function at the molecular level is intricate. Several years ago, we created a comprehensive inventory of all vesicle components. Because vesicles are tiny and complex in composition, this undertaking was not easy. Several teams from Germany, Japan, Switzerland and the United States collaborated for years in order to identify the protein and fat components of vesicles and develop a quantitative molecular model of a standard vesicle.
First, problems had to be solved that were not as straightforward as one might suppose, for example counting the number of vesicles in a solution and determining the quantities of proteins and membrane lipids present. The results were surprising even for experts. It turned out that the structure of a biological transport vesicle is shaped to a far greater extent by proteins than was previously thought: If the vesicle model is looked at from the outside, the lipid membrane (yellow) can barely be seen for the sheer number of proteins. Yet the model only contains around 70 percent of the total quantity of protein present.
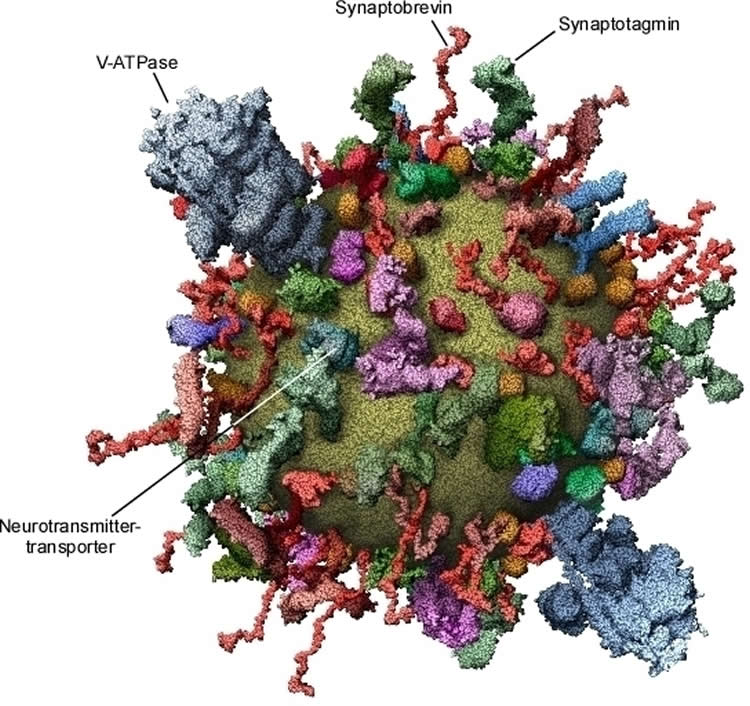
This work formed the basis for further investigations. It has meanwhile been possible to distinguish the vesicles that transport various neurotransmitters from each other and to compare them. Contrary to what was believed, they differ only slightly in their composition. In addition, various vesicles that had already docked to the plasma membrane were isolated, allowing their protein composition to be analyzed as well.
How do the membranes fuse?
A second aim of the research is to gain an understanding of the functional details of the protein machine responsible for membrane fusion. The proteins involved have been characterized for over a decade, but is still not clear how they manage to fuse the membranes within less than one millisecond following an influx of calcium ions.
SNARE proteins – small protein molecules that reside in the plasma membrane and vesicle membrane − are responsible for the fusion process itself. Whenever the membranes approach each other, the proteins stack on top of each other, twisting together like ropes in the direction of the membrane.
This stacking process releases energy, which is used for fusing the membranes. Recent studies have shown that the twisted bundles extend into the membrane.
To understand how this stacking causes the membranes to fuse, the SNARE proteins were incorporated into artificial membranes, which could be observed using high-resolution methods such as cryo-electron microscopy. For the first time, intermediate stages of the fusion reaction were identified. This made it possible to develop a model of the individual steps at the molecular level.
Progress has also been achieved regarding the question of how the inflowing calcium ions activate the fusion machine. This is mediated by another protein in the membrane of synaptic vesicles, synaptotagmin, which binds the calcium ions and then draws the membranes closer together.
Despite considerable progress, the complex molecular processes are still not fully understood. It is all the more astonishing how smoothly neurons communicate with each other and how effectively the fusion machinery works in the synapse to effect our every movement, emotion and thought. Scientists the world over therefore continue to focus on the fusion machine in order to gain a better understanding of these processes. There is justified hope that such knowledge will also improve our understanding of neurodegenerative diseases such as Parkinson’s, Alzheimer’s and Huntington’s disease.
About this neuroscience research article
Source: Reinhard Jahn – Max Planck Institute
Image Source: NeuroscienceNews.com images are credited to MPI for Biophysical Chemistry.
Cite This NeuroscienceNews.com Article
Max Planck Institute. “How Neurons Talk to Each Other.” NeuroscienceNews. NeuroscienceNews, 24 September 2016.