Summary: Using human retinal tissue grown from stem cells, researchers shed light on how color vision develops. The study found thyroid hormones dictated whether the cells became blue, green or red photoreceptors.
Source: Johns Hopkins University.
Biologists at Johns Hopkins University grew human retinas from scratch to determine how cells that allow people to see in color are made.
The work, set for publication in the journal Science, lays the foundation to develop therapies for eye diseases such as color blindness and macular degeneration. It also establishes lab-created “organoids” as a model to study human development on a cellular level.
“Everything we examine looks like a normal developing eye, just growing in a dish,” said Robert Johnston, a developmental biologist at Johns Hopkins. “You have a model system that you can manipulate without studying humans directly.”
Johnston’s lab explores how a cell’s fate is determined—or what happens in the womb to turn a developing cell into a specific type of cell, an aspect of human biology that is largely unknown.
Here, he and his team focused on the cells that allow people to see blue, red and green—the three cone photoreceptors in the human eye.
While most vision research is done on mice and fish, neither of those species has the dynamic daytime and color vision of humans. So Johnston’s team created the human eye tissue they needed—with stem cells.
“Trichromatic color vision differentiates us from most other mammals,” said lead author Kiara Eldred, a Johns Hopkins graduate student. “Our research is really trying to figure out what pathways these cells take to give us that special color vision.”
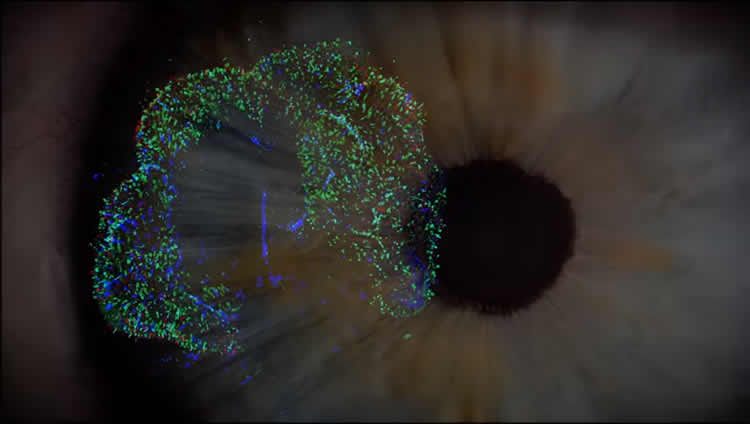
Over months, as the cells grew in the lab and became full-blown retina tissue, the team found the blue-detecting cells materialized first, followed by the red- and green-detecting ones. In both cases, they found the key to the molecular switch was the ebb and flow of thyroid hormone. Importantly, the level of this hormone wasn’t controlled by the thyroid gland, which of course isn’t in the dish, but entirely by the eye itself.
Understanding how the amount of thyroid hormone dictated whether the cells became blue or red and green receptors, the team was able to manipulate the outcome, creating retinas that if they were part of a complete human eye would only see blue, and ones that could only detect green and red.
The finding that thyroid hormone is essential for creating red-green cones provides insight into why pre-term babies, who have lowered thyroid hormone levels as they are lacking the maternal supply, have a higher incidence of vision disorders.
“If we can answer what leads a cell to its terminal fate, we are closer to being able to restore color vision for people who have damaged photoreceptors,” Eldred said. “This is a really beautiful question, both visually and intellectually—what is it that allows us to see color?”
These findings are a first step for the lab. In the future, the researchers would like to use organoids to learn even more about color vision and the mechanisms involved in the creation of other regions of the retina, such as the macula. Since macular degeneration is one of the leading causes of blindness in people, understanding how to grow a new macula could lead to clinical treatments.
“What’s exciting about this is our work establishes human organoids as a model system to study mechanisms of human development,” Johnston said. “What’s really pushing the limit here is that these organoids take nine months to develop just like a human baby. So what we’re really studying is fetal development.”
Source: Jill Rosen – Johns Hopkins University
Publisher: Organized by NeuroscienceNews.com.
Image Source: NeuroscienceNews.com image is adapted from the JHU video.
Video Source: Video credited to JHU.
Original Research: Abstract for “Thyroid hormone signaling specifies cone subtypes in human retinal organoids” by Kiara C. Eldred, Sarah E. Hadyniak, Katarzyna A. Hussey, Boris Brenerman, Ping-Wu Zhang, Xitiz Chamling, Valentin M. Sluch, Derek S. Welsbie, Samer Hattar, James Taylor, Karl Wahlin, Donald J. Zack, and Robert J. Johnston Jr. in Science. Published October 12 2018.
doi:10.1126/science.aau6348
[cbtabs][cbtab title=”MLA”]Johns Hopkins University”Dish Grown Human Retinas Explain How Color Vision Develops.” NeuroscienceNews. NeuroscienceNews, 14 October 2018.
<https://neurosciencenews.com/retina-color-vision-10019/>.[/cbtab][cbtab title=”APA”]Johns Hopkins University(2018, October 14). Dish Grown Human Retinas Explain How Color Vision Develops. NeuroscienceNews. Retrieved October 14, 2018 from https://neurosciencenews.com/retina-color-vision-10019/[/cbtab][cbtab title=”Chicago”]Johns Hopkins University”Dish Grown Human Retinas Explain How Color Vision Develops.” https://neurosciencenews.com/retina-color-vision-10019/ (accessed October 14, 2018).[/cbtab][/cbtabs]
Abstract
Thyroid hormone signaling specifies cone subtypes in human retinal organoids
INTRODUCTION
Cone photoreceptors in the human retina enable daytime, color, and high-acuity vision. The three subtypes of human cones are defined by the visual pigment that they express: blue-opsin (short wavelength; S), green-opsin (medium wavelength; M), or red-opsin (long wavelength; L). Mutations that affect opsin expression or function cause various forms of color blindness and retinal degeneration.
RATIONALE
Our current understanding of the vertebrate eye has been derived primarily from the study of model organisms. We studied the human retina to understand the developmental mechanisms that generate the mosaic of mutually exclusive cone subtypes. Specification of human cones occurs in a two-step process. First, a decision occurs between S versus L/M cone fates. If the L/M fate is chosen, a subsequent choice is made between expression of L- or M-opsin. To determine the mechanism that controls the first decision between S and L/M cone fates, we studied human retinal organoids derived from stem cells.
RESULTS
We found that human organoids and retinas have similar distributions, gene expression profiles, and morphologies of cone subtypes. During development, S cones are specified first, followed by L/M cones. This temporal switch from specification of S cones to generation of L/M cones is controlled by thyroid hormone (TH) signaling. In retinal organoids that lacked thyroid hormone receptor β (Thrβ), all cones developed into the S subtype. Thrβ binds with high affinity to triiodothyronine (T3), the more active form of TH, to regulate gene expression. We observed that addition of T3 early during development induced L/M fate in nearly all cones. Thus, TH signaling through Thrβ is necessary and sufficient to induce L/M cone fate and suppress S fate. TH exists largely in two states: thyroxine (T4), the most abundant circulating form of TH, and T3, which binds TH receptors with high affinity. We hypothesized that the retina itself could modulate TH levels to control subtype fates. We found that deiodinase 3 (DIO3), an enzyme that degrades both T3 and T4, was expressed early in organoid and retina development. Conversely, deiodinase 2 (DIO2), an enzyme that converts T4 to active T3, as well as TH carriers and transporters, were expressed later in development. Temporally dynamic expression of TH-degrading and -activating proteins supports a model in which the retina itself controls TH levels, ensuring low TH signaling early to specify S cones and high TH signaling later in development to produce L/M cones.
CONCLUSION
Studies of model organisms and human epidemiology often generate hypotheses about human biology that cannot be studied in humans. Organoids provide a system to determine the mechanisms of human development, enabling direct testing of hypotheses in developing human tissue. Our studies identify temporal regulation of TH signaling as a mechanism that controls cone subtype specification in humans. Consistent with our findings, preterm human infants with low T3 and T4 have an increased incidence of color vision defects. Moreover, our identification of a mechanism that generates one cone subtype while suppressing the other, coupled with successful transplantation and incorporation of stem cell–derived photoreceptors in mice, suggests that the promise of therapies to treat human diseases such as color blindness, retinitis pigmentosa, and macular degeneration will be achieved in the near future.