Research reported today by Tufts University biologists shows for the first time that bioelectrical signals among cells control and instruct embryonic brain development and manipulating these signals can repair genetic defects and induce development of healthy brain tissue in locations where it would not ordinarily grow.
The research reveals that bioelectric signaling regulates the activity of two cell reprogramming factors (proteins that can turn adult cells into stem cells), which for the first time were analyzed in Xenopus laevis embryos, which share many evolutionary traits with humans. Results appear in the March 11, 2015, edition of the Journal of Neuroscience.
“We’ve found that cells communicate, even across long distances in the embryo, using bioelectrical signals, and they use this information to know where to form a brain and how big that brain should be,” says the paper’s corresponding author Michael Levin, Ph.D., who holds the Vannevar Bush Chair in biology and directs the Center for Regenerative and Developmental Biology in the School of Arts and Sciences at Tufts. “The signals are not just necessary for normal development; they are instructive.”
Levin uses an analogy to a computer. “Bioelectrical signals are not simply the switch that turns the computer on or off, passively allowing it to perform its functions. They actually carry important information, functioning like the software that enables the computer to carry out complex activities.”
These bioelectric signals are implemented by changes in the voltage difference across cell membranes – called the cellular resting potential — and the patterns of differential voltages across anatomical regions.
Bioelectric signaling involves different cell types, including mature somatic cells and stem cells. Prior work in the Levin lab revealed roles for bioelectric gradients in eye, limb and visceral organ patterning, and the new paper found that natural embryonic voltage gradients instruct the formation of the brain.
Overriding Genetic Defects
“This latest research also demonstrated molecular techniques for ‘hijacking’ this bioelectric communication to force the body to make new brain tissue at other locations and to fix genetic defects that cause brain malformation,” says Levin. “This means we may be able to induce growth of new brain tissue to address birth defects or brain injury, which is very exciting for regenerative medicine.”
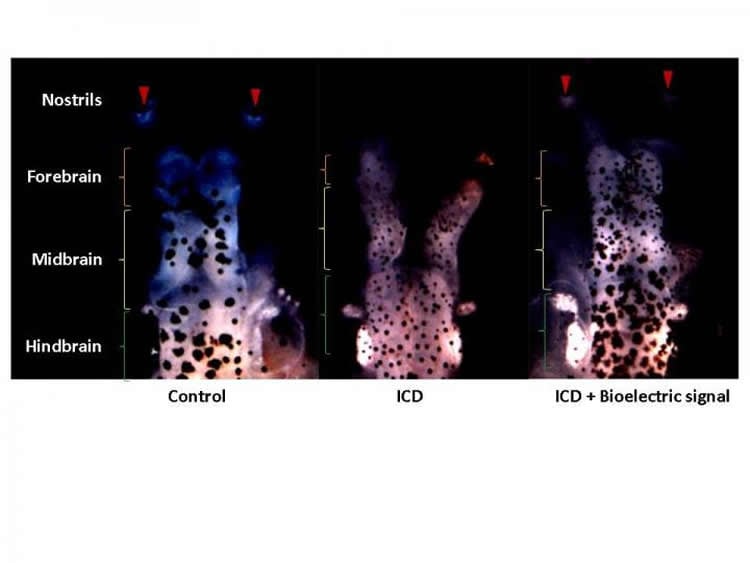
A case in point is the Notch signaling pathway, a protein signaling system that plays a role in neural cell growth and differentiation in mammals and most other multicellular organisms. Defects in Notch signaling disrupt brain development and are also associated with disorders such as T-cell acute lymphoblastic leukemia and multiple sclerosis. The research team found that using molecular techniques to force proper bioelectrical states in cells enabled them to override the defects induced by Notch malfunction, resulting in a much more normal brain despite a genetically-defective Notch protein.
“Using bioelectric signals to control tissue shape doesn’t require micromanaging the genetics of the cells, which can have severe adverse effects,” notes Vaibhav Pai, Ph.D., first author on the paper and research associate in the Levin lab.
Bioelectricity and reprogramming factors work together to regulate tissue fate, says Levin. “Additional study is needed to understand more fully the mechanisms by which electric signaling interacts with genetic networks. However, here we show two such steps, involving calcium signaling and cell-cell communication via electrical synapses known as gap junctions. We are working on applying these techniques in biomedical contexts, especially ion channel modulating drugs–electroceuticals–to repair defects and induce brain regeneration.”
Additional authors on the paper are Joan M. Lemire, and Jean-Francois Pare, research associates in the Department of Biology and Center for Regenerative and Development Biology at Tufts, and Gufa Lin and Ying Chen, of the Stem Cell Institute at the University of Minnesota.
Research reported in this release was supported by the National Institutes of Health under award numbers AR055993-01 and 1R01HD081326-01, the National Science Foundation under award number CBET-0939511 and the G. Harold and Leila Y. Mathers Charitable Foundation.
Contact: Kim Thurler – Tufts University
Source: Tufts University press release
Image Source: The image is credited to Tufts University and is adapted from the press release
Original Research: Abstract for “Endogenous Gradients of Resting Potential Instructively Pattern Embryonic Neural Tissue via Notch Signaling and Regulation of Proliferation” by Vaibhav P. Pai, Joan M. Lemire, Jean-François Paré, Gufa Lin, Ying Chen, and Michael Levin in Journal of Neuroscience. Published online March 11 2015 doi:10.1523/JNEUROSCI.1877-14.2015