Researchers at the University of Wisconsin School of Medicine and Public Health were interested in seeing how different members of a family of proteins affect the speed of electrical signals passed from one brain cell to the next.
The change in speed affects the rate at which neurons talk to each other, which likely has implications in how entire ‘circuits’ of cells communicate.
So they did a little engineering. Normally, neuron-to-neuron communication is controlled by members of the synaptotagmin protein family. The proteins are attached to vesicles—small bundles in the neuron that contain neurotransmitters—and help the vesicle release its cargo into the synapse, transmitting a signal to another cell.
The speed of this release determines, among other things, how quickly and how strongly the receiving neuron can respond to the next wave of signals.
Two recent papers describe how researchers engineered chimeras, proteins built in a cut-and-paste fashion from two existing proteins, to “tune” the speed of neurotransmitter release. One study used parts from the fastest and slowest members of the Synaptotagmin family (syt1 and syt7).
The work was conducted by UW-Madison graduate students Chantell Evans, who performed the biochemistry, and David Ruhl, who performed the experiments in cells. A second study, performed by postdoctoral fellows Renhao Xue and Jon Gaffaney, used syt1 and a calcium-binding protein found in the brain and other various tissues, Doc2B.
To measure the speed (or kinetics) of neuron-to-neuron communication, the researchers grew brain cells in dishes, stimulated individual neurons, and recorded the electrical current as it flows into the receiving neuron over time.
One neuron firing looks somewhat like a heart monitor measuring a heartbeat, but with only one very fast spike in activity and a decaying tail with an exponential curve. But with the chimeras, the spike of activity was slower to reach its pinnacle and the tail took longer to return to a resting state as well.
“When we put these chimeras into neurons, it slowed the rise of the postsynaptic currents and it also slowed their decays,” said Ed Chapman, a Howard Hughes Medical Institute investigator and professor of neuroscience in the School of Medicine and Public Health. “We were able to change the speed of the synapse by changing the intrinsic speed of the calcium sensor that drives vesicle exocytosis.”
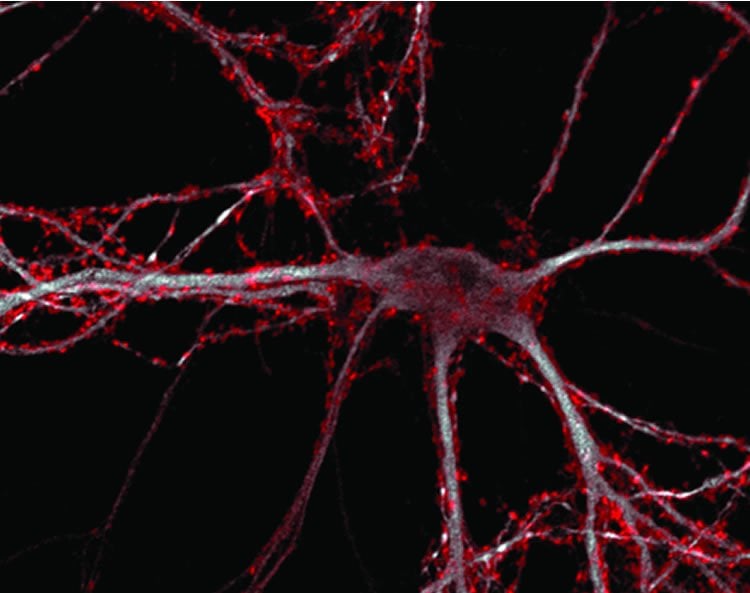
The rate of that decay is a big deal, as it underlies the ability of neuronal circuits to “reverberate,” that is, to continue to signal one-another for a prolonged period of time, and this is thought to mediate certain forms of short-term memory. By changing the speed of the synaptic decays, Chapman and co-workers can now conduct experiments to determine the rules that underlie reverberation.
Going forward, the Chapman lab is taking its electrophysiology work down the hall to Bob Pearce, chair of anesthesiology at the School of Medicine and Public Health, to put these chimeras into the brains of mice to examine their effects on the function of an entire network, and ultimately to look at differences in cognition and behavior.
Additional Information: NeuroscienceNews.com would like to thank David Ruhl for submitting this article to us. David Ruhl is a National Science Foundation GRFP Fellow and part of the University of Wisconsin Madision Neuroscience Training Program. He is also a researcher at the Lab of Edwin Chapman, Howard Hughes Medical Institute.
Source: David Ruhl – University of Wisconsin Madision
Image Credit: The image is credited to Evans, Ruhl et al./Journal of Neuroscience
Original Research: Abstract for “An Engineered Metal Sensor Tunes the Kinetics of Synaptic Transmission” by Chantell S. Evans, David A. Ruhl, and Edwin R. Chapman in Journal of Neuroscience. Published online August 26 2015 doi:10.1523/JNEUROSCI.1694-15.2015
Abstract for “Structural elements that underlie Doc2β function during asynchronous synaptic transmission” by Renhao Xue, Jon D. Gaffaney, and Edwin R. Chapman in PNAS. Published online August 4 2015 doi:10.1073/pnas.1502288112
Abstract
An Engineered Metal Sensor Tunes the Kinetics of Synaptic Transmission
The Ca2+ sensor synaptotagmin-1 (syt-1) regulates neurotransmitter release by interacting with anionic phospholipids. Here we test the idea that the intrinsic kinetics of syt–membrane interactions determine, in part, the time course of synaptic transmission. To tune the kinetics of this interaction, we grafted structural elements from the slowest isoform, syt-7, onto the fastest isoform, syt-1, resulting in a chimera with intermediate kinetic properties. Moreover, the chimera coupled a physiologically irrelevant metal, Sr2+, to membrane fusion in vitro. When substituted for syt-1 in mouse hippocampal neurons, the chimera slowed the kinetics of synaptic transmission. Neurons expressing the chimera also evinced rapid and efficient Sr2+ triggered release, in contrast to the weak response of neurons expressing syt-1. These findings reveal presynaptic sensor–membrane interactions as a major factor regulating the speed of the release machinery. Finally, the chimera failed to clamp the elevated spontaneous fusion rate exhibited by syt-1 KO neurons, indicating that the metal binding loops of syt-1 regulate the two modes of release by distinct mechanisms.
SIGNIFICANCE STATEMENT In calcium, synaptotagmin-1 triggers neurotransmitter release by interacting with membranes. Here, we demonstrate that intrinsic properties of this interaction control the time course of synaptic transmission. We engineered a “chimera” using synaptotagmin-1 and elements of a slower isoform, synaptotagmin-7. When expressed in neurons, the chimera slowed the rate of neurotransmitter release. Furthermore, unlike native synaptotagmin-1, the chimera was able to function robustly in the presence of strontium–a metal not present in cells. We exploited this ability to show that a key function of synaptotagmin-1 is to penetrate cell membranes. This work sheds light on fundamental mechanisms of neurotransmitter release.
“An Engineered Metal Sensor Tunes the Kinetics of Synaptic Transmission” by Chantell S. Evans, David A. Ruhl, and Edwin R. Chapman in Journal of Neuroscience. Published online August 26 2015 doi:10.1523/JNEUROSCI.1694-15.2015
Abstract
Structural elements that underlie Doc2β function during asynchronous synaptic transmission
Double C2-like domain-containing proteins alpha and beta (Doc2α and Doc2β) are tandem C2-domain proteins proposed to function as Ca2+ sensors for asynchronous neurotransmitter release. Here, we systematically analyze each of the negatively charged residues that mediate binding of Ca2+ to the β isoform. The Ca2+ ligands in the C2A domain were dispensable for Ca2+-dependent translocation to the plasma membrane, with one exception: neutralization of D220 resulted in constitutive translocation. In contrast, three of the five Ca2+ ligands in the C2B domain are required for translocation. Importantly, translocation was correlated with the ability of the mutants to enhance asynchronous release when overexpressed in neurons. Finally, replacement of specific Ca2+/lipid-binding loops of synaptotagmin 1, a Ca2+ sensor for synchronous release, with corresponding loops from Doc2β, resulted in chimeras that yielded slower kinetics in vitro and slower excitatory postsynaptic current decays in neurons. Together, these data reveal the key determinants of Doc2β that underlie its function during the slow phase of synaptic transmission.
“Structural elements that underlie Doc2β function during asynchronous synaptic transmission” by Renhao Xue, Jon D. Gaffaney, and Edwin R. Chapman in PNAS. Published online August 4 2015 doi:10.1073/pnas.1502288112