Summary: A new study reveals how calcium ion channels in the brain don’t just relay signals—they remember them. Researchers discovered that CaV2.1 channels at synapses can adopt nearly 200 shapes based on electrical activity, effectively entering a “memory state” that weakens signal transmission.
These brief molecular memories accumulate over time, leading to long-term synaptic changes essential for learning and memory formation. Like a clutch in a car, a part of the channel disengages after repeated activity, preventing further signal flow and reshaping communication between neurons.
Key Facts:
- Molecular Memory: CaV2.1 calcium channels “remember” prior activity by changing shape and temporarily blocking signal flow.
- Synaptic Plasticity Link: Short-term channel memory accumulates, driving long-term synaptic changes.
- Drug Targeting Potential: The study pinpoints specific parts of the channel that could be targeted to treat neurological disorders.
Source: Linkoping University
One of the brain’s superpowers is its ability to learn from past experiences and form memories. These vital processes depend on the reshaping of connections between neurons in the brain.
Nerve junctions, called synapses, are strengthened or weakened throughout life in such a way that the brain is, in a certain sense, constantly being reshaped at the cellular level.
This phenomenon is called synaptic plasticity.
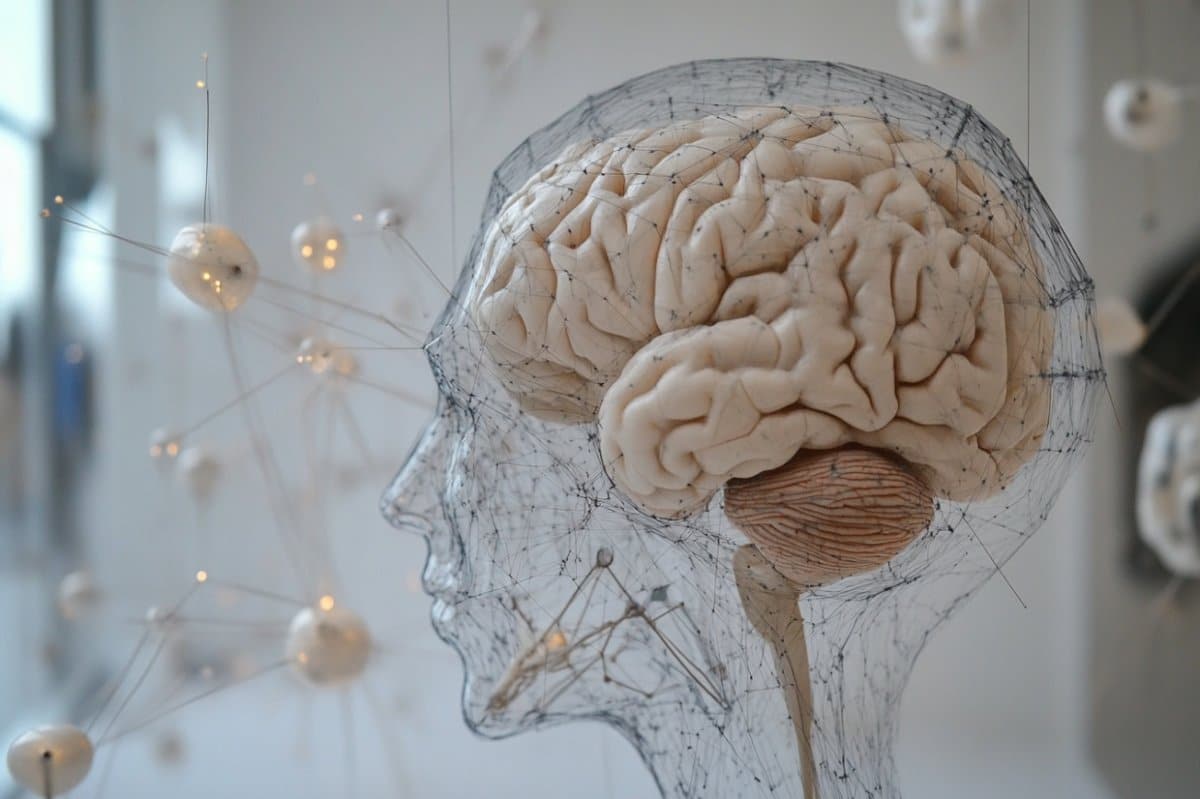
There are several processes contributing to synaptic plasticity in the nervous system. One of these processes has to do with a type of molecules called calcium ion channels, which have long been of interest to researchers at Linköping University (LiU).
“I want to uncover the secret lives of these ion channel molecules. Calcium ion channels have very important functions in the body – by opening and closing, they regulate, among other things, nerve-to-nerve signalling.
But beyond that, these molecules also have a kind of memory of their own, and can remember previous nerve signals,” says Antonios Pantazis, associate professor at the Department of Biomedical and Clinical Sciences at LiU, who led the study.
The focus of this study was on a specific type of ion channel, the CaV2.1 channel, which is the most common calcium ion channel in the brain. The ion channel is located at the synapse, at the very end of the neuron.
When an electrical signal passes through the neuron, the ion channel open, setting in motion a process leading to neurotransmitter being released towards the receiving neuron in the synapse. In this way, CaV2.1 channels are the gatekeepers of synaptic, neuron-to-neuron communication.
Prolonged electrical activity reduces the number of CaV2.1 channels that can open, resulting in less transmitter release, so the receiving neuron receives a weaker message.
It is as if the channels can ‘remember’ previous signalling, and in doing so, make themselves unavailable to open by subsequent signals. How this works at the molecular level has been unknown to scientists until now.
The Linköping researchers have now discovered a mechanism for how the ion channel can ‘remember’. The channel is a large molecule made up of several interconnected parts, which can move relative to each other in response to electrical signals.
They discovered that the ion channel can take almost 200 different shapes depending on the strength and duration of an electrical signal; it is a very complex molecular machine.
“We believe that during sustained electrical nerve signalling, an important part of the molecule disconnects from the channel gate, similar to the way the clutch in a car breaks the connection between the engine and the wheels.
“The ion channel can then no longer be opened. When hundreds of signals occur over long enough time, they can convert most channels into this ‘declutched memory state’ for several seconds,” says Antonios Pantazis.
If the ion channel can ‘remember’ for just a few seconds, how does it contribute to lifelong learning? This type of collective memory in the ion channels can accumulate over time and reduce the communication between two neurons.
This then leads to changes in the receiving neuron, lasting for hours or days. Eventually, it results in much longer-lived changes in the brain, such as the elimination of weakened synapses.
“In this way, a ‘memory’ that lasts for a few seconds in a single molecule can make a small contribution to a person’s memory that lasts for a lifetime,” says Antonios Pantazis.
Increased knowledge of how these calcium ion channels work can in the long term contribute to the treatment of certain diseases. There are many variants of the gene that produces the CaV2.1 channel, CACNA1A, that are linked to rare but serious neurological diseases, that often run in families.
To develop drugs against these, it helps to know which part of the large ion channel you want to affect and in what way its activity should be changed.
“Our work pinpoints which part of the protein should be targeted when developing new drugs,” says Antonios Pantazis.
Funding: The research has been funded by the Swedish Research Council, the Linköping University Wallenberg Centre for Molecular Medicine, the Swedish Brain Foundation, the Swedish Heart-Lung Foundation, the Lions Research Fund for Public Diseases and the NIH.
About this neuroscience and memory research news
Author: Antonios Pantazis
Source: Linkoping University
Contact: Antonios Pantazis – Linkoping University
Image: The image is credited to Neuroscience News
Original Research: Open access.
“A rich conformational palette underlies human CaV2.1-channel availability” by Antonios Pantazis et al. Nature Communications
Abstract
A rich conformational palette underlies human CaV2.1-channel availability
Depolarization-evoked opening of CaV2.1 (P/Q-type) Ca2+-channels triggers neurotransmitter release, while voltage-dependent inactivation (VDI) limits channel availability to open, contributing to synaptic plasticity.
The mechanism of CaV2.1 response to voltage is unclear.
Using voltage-clamp fluorometry and kinetic modeling, we optically track and physically characterize the structural dynamics of the four CaV2.1 voltage-sensor domains (VSDs). The VSDs are differentially sensitive to voltage changes, both brief and long-lived.
VSD-I seems to directly drive opening and convert between two modes of function, associated with VDI. VSD-II is apparently voltage-insensitive.
VSD-III and VSD-IV sense more negative voltages and undergo voltage-dependent conversion uncorrelated with VDI. Auxiliary β-subunits regulate VSD-I-to-pore coupling and VSD conversion kinetics.
Hence, the central role of CaV2.1 channels in synaptic release, and their contribution to plasticity, memory formation and learning, can arise from the voltage-dependent conformational changes of VSD-I.