Summary: A new study links hundreds of brain proteins to differences in how brain regions communicate, revealing how microscale molecules can influence macroscale brain connectivity. Researchers studied brain samples and scans from elderly participants to map biochemical mechanisms affecting structural and functional connectivity in the brain.
By integrating protein and RNA data with neuroimaging, they identified key proteins associated with communication between brain areas. Dendritic spines—structures on neurons—served as an important bridge, connecting molecular data with brain-wide networks.
The findings illuminate the complex, multi-level architecture supporting human brain function. This research could pave the way for new approaches in understanding neurodegenerative diseases.
Key Facts:
- Researchers identified proteins linked to individual differences in brain connectivity and structural patterns.
- Dendritic spine morphometry was crucial in linking molecular signals to brain region communication.
- Findings suggest that understanding brain function requires multi-scale data integration across molecules, cells, and brain networks.
Source: University of Alabama
A long-standing goal of neuroscience is to understand how molecules and cellular structures on a microscale give rise to communication between brain regions at the macroscale.
A study published in Nature Neuroscience now identifies, for the first time, hundreds of brain proteins that explain inter-individual differences in functional connectivity and structural covariation in the human brain.
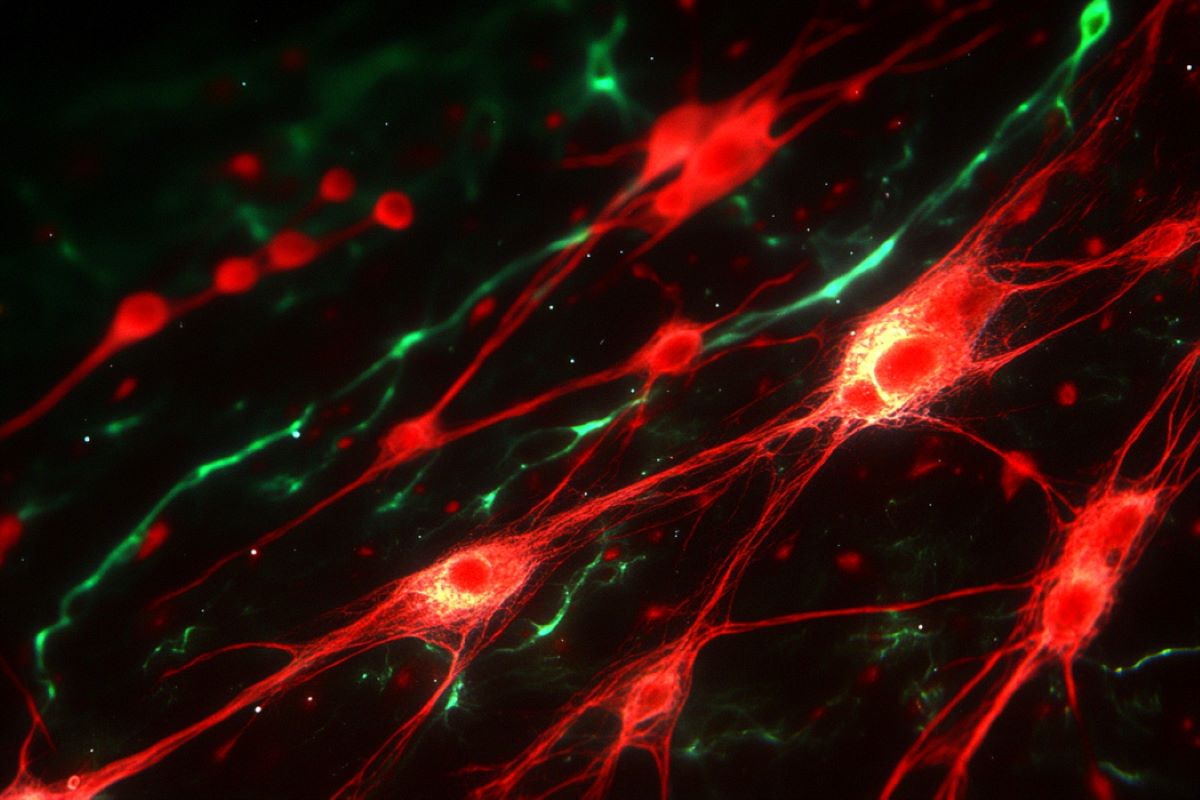
“A central goal of neuroscience is to develop an understanding of the brain that ultimately describes the mechanistic basis of human cognition and behavior,” said Jeremy Herskowitz, Ph.D., associate professor in the University of Alabama at Birmingham Department of Neurology and co-corresponding author of the study with Chris Gaiteri, Ph.D., SUNY Upstate Medical University, Syracuse, New York.
“This study demonstrates the feasibility of integrating data from vastly different biophysical scales to provide a molecular understanding of human brain connectivity.”
Bridging the gap from the molecular scale of proteins and mRNA to the brain-wide neuroimaging scale of functional and structural magnetic resonance imaging — a span of about seven orders of magnitude — was made possible by the Religious Orders Study and Rush Memory and Aging Project, or ROSMAP, at Rush University, Chicago, Illinois.
ROSMAP enrolls Catholic nuns, priests and brothers age 65 or older, who are without known dementia at time of enrollment. Participants receive medical and psychological evaluations each year and agree to donate their brains after death.
Herskowitz, Gaiteri and colleagues studied postmortem brain samples and data from a unique cohort of 98 ROSMAP participants. Their data types included resting state fMRI, structural MRI, genetics, dendritic spine morphometry, proteomics and gene expression measurements from the superior frontal gyrus and inferior temporal gyrus of the brain.
“Based on the stability of functional connectivity patterns within individuals, we hypothesized that it is possible to combine postmortem molecular and subcellular data with antemortem neuroimaging data from the same individuals to prioritize molecular mechanisms underlying brain connectivity,” Herskowitz said.
The average age of the ROSMAP participants at time of MRI scan and at death were 88 +/- 6 years and 91 +/- 6 years, respectively, with an average time interval between the MRI scan and age at death of 3 +/- 2 years.
The average postmortem interval to brain sampling was 8.5 +/- 4.6 hours. In the study, the researchers performed detailed characterization of each omic, cellular and neuroimaging data type, then integrated the different data types using computational clustering algorithms.
The key to the research was using an intermediate scale measurement — dendritic spine morphometry, the shapes, sizes and densities of the spines — to link the molecular scale with the brain-wide neuroimaging scale.
The integration of dendritic spine morphometry to contextualize the proteomic and transcriptomic signals was critical for detecting protein association with functional connectivity.
“Initially, the protein and RNA measures could not explain the person-to-person variability in functional connectivity; however, it all clicked once we integrated the dendritic spine morphology to bridge the gap from molecules to inter-brain region communication,” Herskowitz said.
A dendrite is a branched extension from a neuron body that receives impulses from other neurons. Each dendrite can have thousands of small protrusions called spines. The head of each spine can form a contact point called a synapse to receive an impulse sent from the axon of another neuron.
Dendritic spines can rapidly change shape or volume while forming new synapses, part of the process called brain plasticity, and the head of the spine structurally supports postsynaptic density. Spines can be divided into shape subclasses based on their three-dimensional structure as thin, mushroom, stubby or filopodia.
This summer, in a different study, Herskowitz and colleagues used ROSMAP samples to show that preservation of memory in the very old was maintained by the quality, as measured by dendritic spine head diameter, not the quantity of synapses in the brain.
In this latest study, the hundreds of proteins the researchers identified that explain inter-individual differences in functional connectivity and structural covariation were enriched for proteins involved in synapses, energy metabolism and RNA processing.
“By integrating data at the genetic, molecular, subcellular and tissue levels, we linked specific biochemical changes at synapses to connectivity between brain regions,” Herskowitz said.
“Overall, this study indicates that acquiring data across the major perspectives in human neuroscience from the same set of brains is foundational for understanding how human brain function is supported at multiple biophysical scales,” Herskowitz said.
“While future research is necessary for fully determining the scope and components of multi-scale brain synchrony, we have established a robustly defined initial set of molecules whose effects likely resonate across biophysical scales.”
Besides Herskowitz and Gaiteri, co-authors of the study, “Multiscale Integration Identifies Synaptic Proteins Associated with Human Brain Connectivity,” are Bernard Ng, Shinya Tasaki and David A. Bennett, Rush University Medical Center, Chicago, Illinois; Kelsey M. Greathouse, Courtney K. Walker, Audrey J. Weber, Ashley B. Adamson, Julia P. Andrade, Emily H. Poovey, Kendall A. Curtis and Hamad M. Muhammad, UAB Department of Neurology and Center for Neurodegeneration and Experimental Therapeutics; Ada Zhang, SUNY Upstate Medical University; Sydney Covitz, Matt Cieslak, Jakob Seidlitz, Ted Satterthwaite and Jacob Vogel, University of Pennsylvania, Philadelphia, Pennsylvania; and Nicholas T. Seyfried, Emory University School of Medicine, Atlanta, Georgia.
Funding: Support came from National Institutes of Health grants AG061800, AG061798, AG057911, AG067635, AG054719, AG063755, AG068024, NS061788, AG10161, AG72975, AG15819, AG17917, AG46152 and AG61356.
About this neuroscience and genetics research news
Author: Jeffrey Hansen
Source: University of Alabama
Contact: Jeffrey Hansen – University of Alabama
Image: The image is credited to Neuroscience News
Original Research: Open access.
“Integration across biophysical scales identifies molecular and cellular correlates of person-to-person variability in human brain connectivity” by Jeremy Herskowitz et al. Nature Neuroscience
Abstract
Integration across biophysical scales identifies molecular and cellular correlates of person-to-person variability in human brain connectivity
Brain connectivity arises from interactions across biophysical scales, ranging from molecular to cellular to anatomical to network level. To date, there has been little progress toward integrated analysis across these scales.
To bridge this gap, from a unique cohort of 98 individuals, we collected antemortem neuroimaging and genetic data, as well as postmortem dendritic spine morphometric, proteomic and gene expression data from the superior frontal and inferior temporal gyri.
Through the integration of the molecular and dendritic spine morphology data, we identified hundreds of proteins that explain interindividual differences in functional connectivity and structural variation.
These proteins are enriched for synaptic structures and functions, energy metabolism and RNA processing. By integrating data at the genetic, molecular, subcellular and tissue levels, we link specific biochemical changes at synapses to connectivity between brain regions.
These results demonstrate the feasibility of integrating data from vastly different biophysical scales to provide a more comprehensive understanding of brain connectivity.