Summary: Researchers have developed lab-grown neurons that behave more like real brain networks, advancing the study of learning and memory. Using microfluidic devices, the neurons formed diverse and functional networks, resembling those seen in living nervous systems.
These networks exhibited complex activity patterns and showed neural plasticity, reconfiguring in response to repetitive stimulation. This breakthrough provides scientists with a powerful new tool to study brain functions under controlled lab conditions.
Key Facts
- Realistic Networks: Microfluidic devices enabled neurons to form natural-like brain networks.
- Neural Plasticity: Repeated stimulation altered neuronal ensembles, mimicking learning processes.
- Advanced Models: This technology can be used to study memory formation and brain function.
Source: Tohoku University
“Neurons that fire together, wire together” describes the neural plasticity seen in human brains, but neurons grown in a dish don’t seem to follow these rules. Neurons that are cultured in-vitro form random and meaningless networks that all fire together. They don’t accurately represent how a real brain would learn, so we can only draw limited conclusions from studying it.
But what if we could develop in-vitro neurons that actually behaved more naturally?
A research team at Tohoku University has used microfluidic devices to reconstitute biological neuronal networks bearing connectivity resembling that found in animal nervous systems.
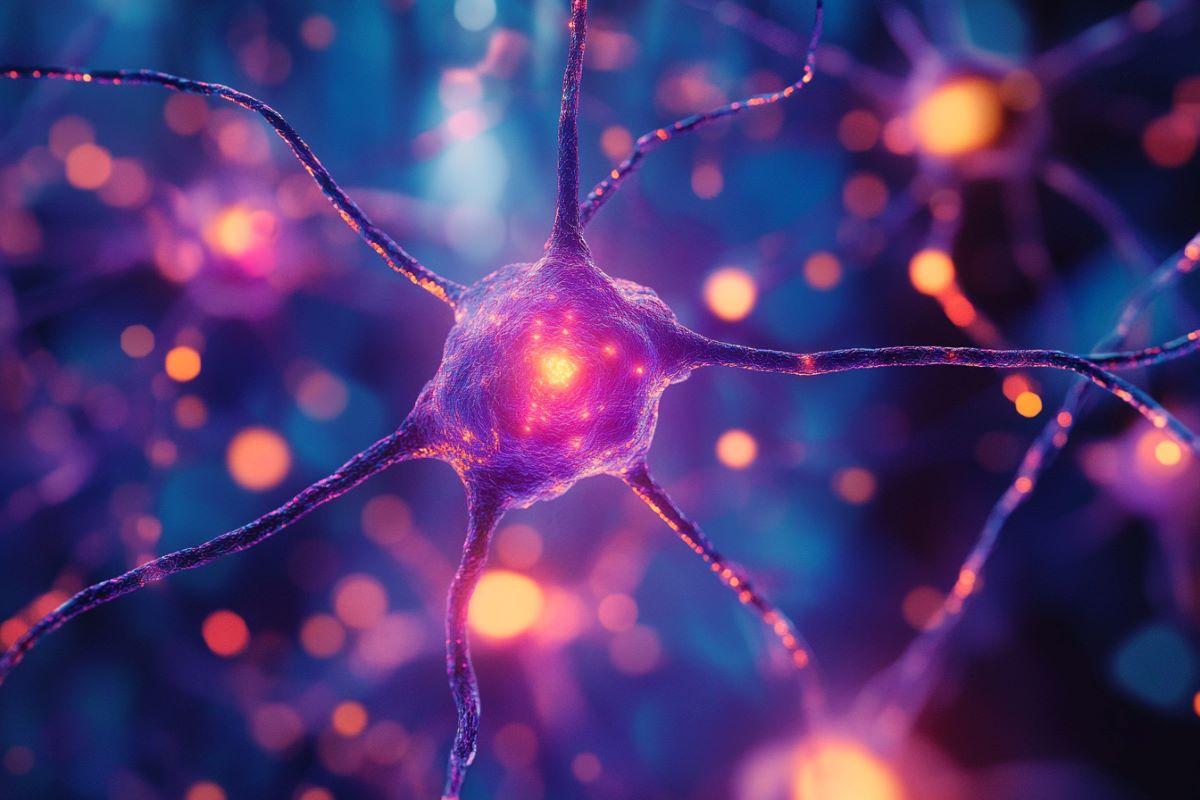
They showed that such networks exhibit complex activity patterns that were able to be “reconfigured” by repetitive stimulation. This remarkable finding provides new tools for studying learning and memory.
The results were published online in Advanced Materials Technologies on November 23, 2024.
In certain areas of the brain, information is encoded and stored as “neuronal ensembles,” or groups of neurons that fire together. Ensembles change based on input signals from the environment, which is considered to be the neural basis of how we learn and remember things. However, studying these processes using animal models is difficult because of its complex structure.
“The reason there is a need to grow neurons in the lab is because the systems are much simpler,” remarks Hideaki Yamamoto (Tohoku University), “Lab-grown neurons allow scientists to explore how learning and memory work in highly controlled conditions. There is a demand for these neurons to be as close to the real thing as possible.”
The research team created a special model using a microfluidic device–a small chip with tiny 3D structures. This device allowed neurons to connect and form networks similar to those in the animals’ nervous system. By changing the size and shape of the tiny tunnels (called microchannels) that connect the neurons, the team controlled how strongly the neurons interacted.
The researchers demonstrated that networks with smaller microchannels can maintain diverse neuronal ensembles. For example, the in-vitro neurons grown in traditional devices tended to only exhibit a single ensemble, while those grown with the smaller microchannels showed up to six ensembles.
Additionally, the team found that repeated stimulation modulates these ensembles, showing a process resembling neural plasticity, as if the cells were being reconfigured.
This microfluid technology in conjunction with in-vitro neurons could be used in the future to develop more advanced models that can mimic specific brain functions, like forming and recalling memories.
About this neuroplasticity research news
Author: Public Relations
Source: Tohoku University
Contact: Public Relations – Tohoku University
Image: The image is credited to Neuroscience News
Original Research: Open access.
“Precision Microfluidic Control of Neuronal Ensembles in Cultured Cortical Networks” by Hideaki Yamamoto et al. Advanced Materials Technologies
Abstract
Precision Microfluidic Control of Neuronal Ensembles in Cultured Cortical Networks
In vitro neuronal culture is an important research platform in cellular and network neuroscience.
However, neurons cultured on a homogeneous scaffold form dense, randomly connected networks and display excessively synchronized activity; this phenomenon has limited their applications in network-level studies, such as studies of neuronal ensembles, or coordinated activity by a group of neurons.
Herein, polydimethylsiloxane-based microfluidic devices are developed to create small neuronal networks exhibiting a hierarchically modular structure resembling the connectivity observed in the mammalian cortex.
The strength of intermodular coupling is manipulated by varying the width and height of the microchannels that connect the modules.
Neuronal activity recording via calcium imaging shows that the spontaneous activity in networks with smaller microchannels (2.2–5.5 µm2) has lower synchrony and exhibits a threefold variety of neuronal ensembles.
Optogenetic stimulation demonstrates that a reduction in intermodular coupling enriches evoked neuronal activity patterns and that repeated stimulation induces plasticity in neuronal ensembles in these networks.
These findings suggest that cell engineering technologies based on microfluidic devices enable in vitro reconstruction of the intricate dynamics of neuronal ensembles, thus providing a robust platform for studying neuronal ensembles in a well-defined physicochemical environment.