Summary: A new study confirms the age-old adage “practice makes perfect.” Researchers used cutting-edge technology to observe 73,000 neurons in mice as they learned a task. They found that repetitive practice solidifies neural pathways, transforming unstable memory representations into stable ones, leading to improved performance and mastery.
Key Facts:
- Repetitive practice strengthens and stabilizes neural pathways in the brain.
- This “crystallization” of memory circuits improves accuracy and automaticity of learned skills.
- The study used innovative light-beads microscopy to visualize neuronal activity in real time.pen_spark
Source: Rockefeller University
“Practice makes perfect” is no mere cliché, according to a new study from researchers at The Rockefeller University and UCLA. Instead, it’s the recipe for mastering a task, because repeating an activity over and over solidifies neural pathways in your brain.
As they describe in Nature, the scientists used a cutting-edge technology developed by Rockefeller’s Alipasha Vaziri to simultaneously observe 73,000 cortical neurons in mice as the animals learned and repeated a given task over two weeks.
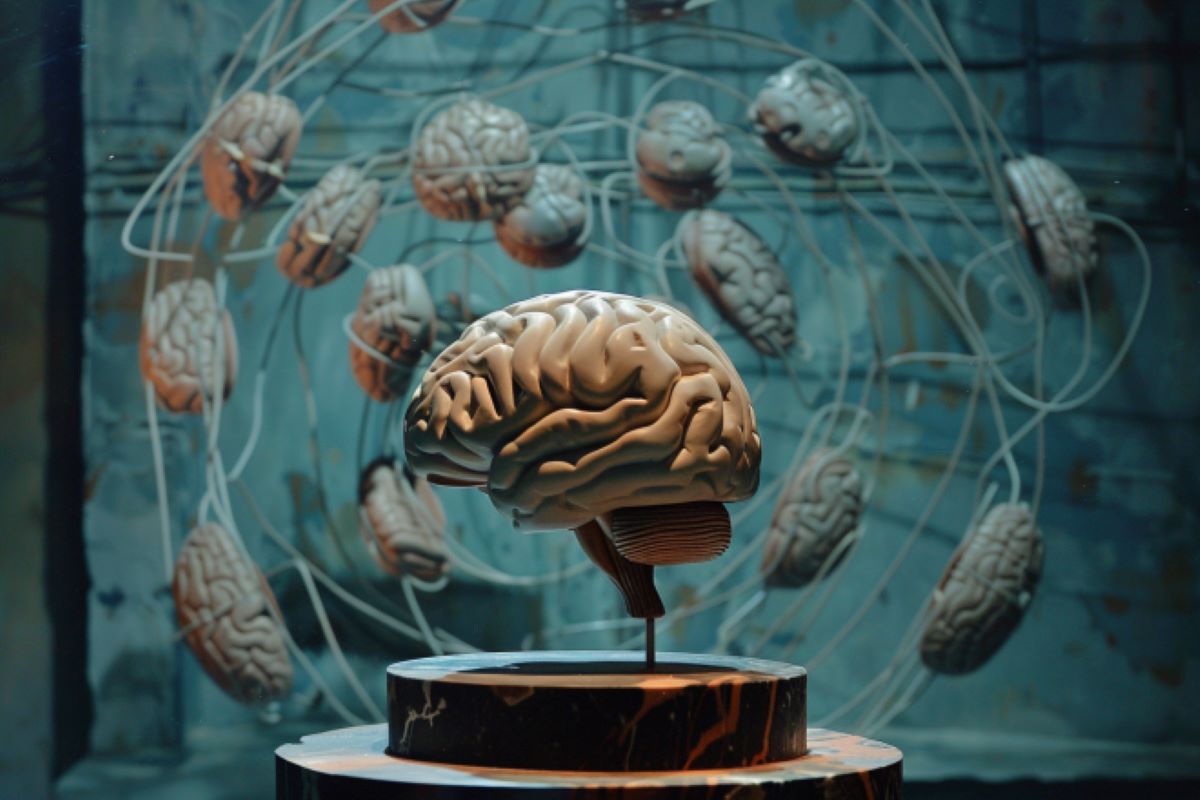
The study revealed that memory representations transform from unstable to solid in working memory circuits, giving insights into why performance becomes more accurate and automatic following repetitive practice.
“In this work we show how working memory—the brain’s ability to hold and process information—improves through practice,” says Vaziri, head of Rockefeller’s Laboratory of Neurology and Biophysics.
“We expect that these insights will not only advance our understanding of learning and memory but also have implications for addressing memory-related disorders.”
Imagining challenges
Working memory is essential to a variety of cognitive functions, and yet the mechanisms underlying memory formation, retention, and recall—which enable us to perform a task we’ve done before without having to learn it anew—remain unclear over long timescales.
For the current study, the researchers wanted to observe the stability of working memory representations over time, and what role these representations played in the ability to skillfully perform the task on cue.
To do so, they sought to record neuronal populations repeatedly in mice over a relatively long period while the animals learned and became experts in a given task.
But they faced a daunting challenge: technical limitations have hampered the ability to image the activity of large population of neurons across the brain in real time, over longer periods, and at any tissue depth in the cortex.
The UCLA researchers turned to Vaziri, who has developed brain imaging techniques that are among the only tools capable of capturing the majority of the mouse cortex in real time at a high resolution and speed.
Vaziri suggested they use light-beads microscopy (LBM), a high-speed volumetric imaging technology he developed that allows for cellular resolution in vivo recording of activity of neuronal populations up to 1 million neurons—a 100-fold increase in the number of neurons that can be simultaneously recorded.
Neural transformations
In the current study, the researchers used LBM to image the cellular activity of 73,000 neurons in mice simultaneously throughout various depths of the cortex and tracked the activity of the same neurons over two weeks as the animals identified, recalled, and repeated a sequence of odors.
They found that the working memory circuits transformed as the mice mastered the proper sequences. Initially, the circuits were unstable, but as the mice practiced the task repeatedly, the circuits began to stabilize and solidify.
“This is what we refer to as ‘crystallization,’” Vaziri says. “The findings essentially illustrate that repetitive training not only enhances skill proficiency but also leads to profound changes in the brain’s memory circuits, making performance more accurate and automatic.”
“If one imagines that each neuron in the brain is sounding a different note, the melody that the brain is generating when it is doing the task was changing from day to day, but then became more and more refined and similar as animals kept practicing the task,” adds corresponding author and UCLA Health neurologist Peyman Golshani.
Crucially, some aspects of these discoveries were uniquely enabled by the large-scale and deep tissue imaging capabilities of LBM. Initially, the researchers used standard two-photon imaging of smaller neuronal populations in upper cortical layers, but they failed to find evidence for memory stabilization.
But once they employed LBM to record from over 70,000 neurons in deeper cortical regions, they were able to observe the crystallization of working memory representations that accompanied the mice’s increasing mastery of the task.
“In the future, we may tackle the role of different neuronal cell types involved in mediating this mechanism, and in particular the interaction of different types of interneurons with excitatory cells,” Vaziri says.
“We’re also interested in understanding how learning is implemented and could be transferred into a new context—that is, how the brain could generalize from a learned task to some new unknown problems.”
About this memory research news
Author: Katherine Fenz
Source: Rockefeller University
Contact: Katherine Fenz – Rockefeller University
Image: The image is credited to Neuroscience News
Original Research: Open access.
“Volatile working memory representations crystallize with practice” by Alipasha Vaziri et al. Nature
Abstract
Volatile working memory representations crystallize with practice
Working memory, the process through which information is transiently maintained and manipulated over a brief period, is essential for most cognitive functions.
However, the mechanisms underlying the generation and evolution of working-memory neuronal representations at the population level over long timescales remain unclear.
Here, to identify these mechanisms, we trained head-fixed mice to perform an olfactory delayed-association task in which the mice made decisions depending on the sequential identity of two odours separated by a 5 s delay.
Optogenetic inhibition of secondary motor neurons during the late-delay and choice epochs strongly impaired the task performance of the mice.
Mesoscopic calcium imaging of large neuronal populations of the secondary motor cortex (M2), retrosplenial cortex (RSA) and primary motor cortex (M1) showed that many late-delay-epoch-selective neurons emerged in M2 as the mice learned the task.
Working-memory late-delay decoding accuracy substantially improved in the M2, but not in the M1 or RSA, as the mice became experts.
During the early expert phase, working-memory representations during the late-delay epoch drifted across days, while the stimulus and choice representations stabilized.
In contrast to single-plane layer 2/3 (L2/3) imaging, simultaneous volumetric calcium imaging of up to 73,307 M2 neurons, which included superficial L5 neurons, also revealed stabilization of late-delay working-memory representations with continued practice.
Thus, delay- and choice-related activities that are essential for working-memory performance drift during learning and stabilize only after several days of expert performance.