Summary: Researchers have uncovered a groundbreaking mechanism called Electro-Calcium (E-Ca) coupling that integrates electrical and calcium signaling in brain capillaries. This process ensures precise blood flow delivery to active neurons, crucial for brain health and cognitive function.
Using advanced imaging, they demonstrated how electrical waves amplify calcium activity, fine-tuning blood flow across the brain’s capillary network. This discovery could pave the way for therapies targeting neurological diseases like Alzheimer’s by restoring disrupted blood flow.
Key Facts:
- Electro-Calcium Coupling: Integrates electrical and calcium signals to regulate blood flow in brain capillaries.
- Improved Blood Flow: Electrical signals boost calcium activity by 76%, enhancing capillary network synchronization.
- Therapeutic Potential: Offers insights for treating neurological diseases like Alzheimer’s by restoring blood flow.
Source: University of Vermont
A team of UVM scientists led by Mark Nelson, Ph.D., from the Larner College of Medicine at the University of Vermont, has uncovered a novel mechanism that reshapes our understanding of how blood flow is regulated in the brain.
The study, published in The Proceedings of the National Academy of Sciences (PNAS), a peer reviewed journal of the National Academy of Sciences (NAS), introduces Electro-Calcium (E-Ca) Coupling, a process that integrates electrical and calcium signaling in brain capillaries to ensure precise blood flow delivery to active neurons.
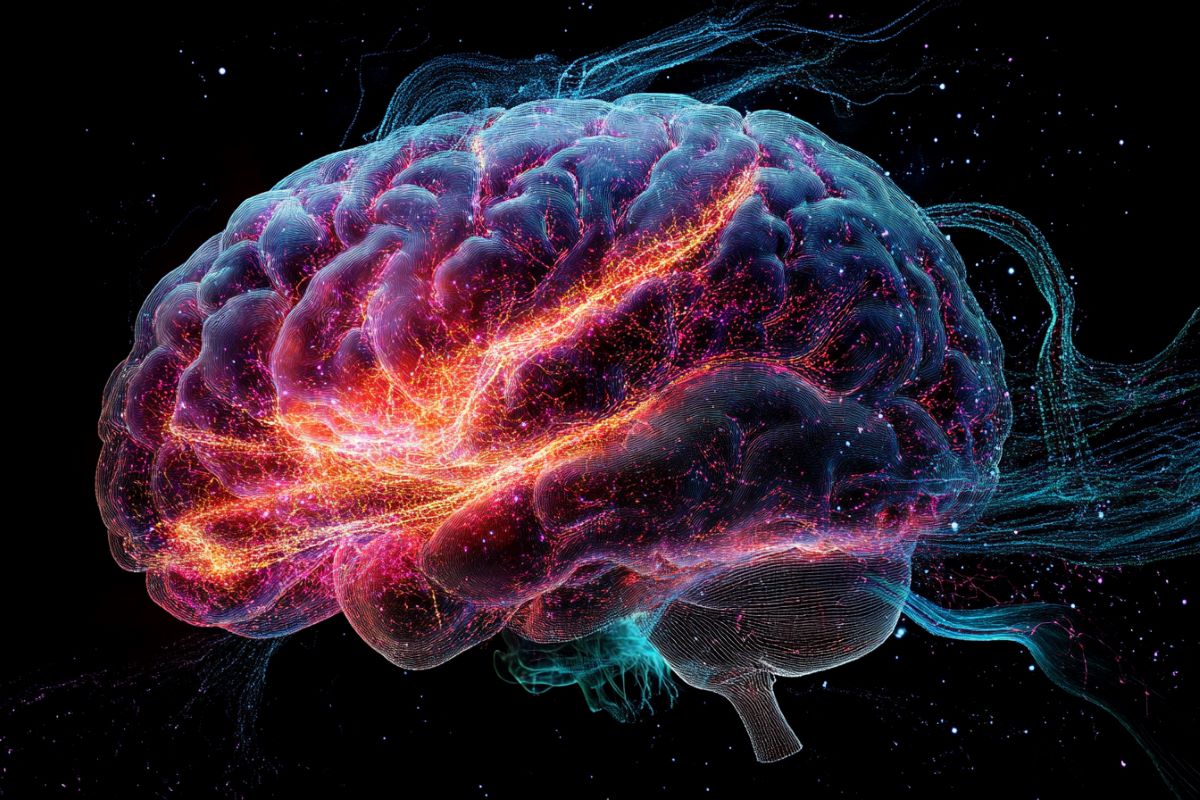
In the human body, blood is delivered into the brain from surface arteries through penetrating arterioles, or very small blood vessel that branch off from arteries, and hundreds of miles of capillaries, which enormously extend the territory of perfusion.
The brain—a highly metabolically demanding organ that lacks substantial energy reserves—maintains constant blood flow in the face of blood pressure fluctuations (autoregulation) but relies on an on-demand delivery process in which neuronal activity triggers a local increase in blood flow to selectively distribute oxygen and nutrients to active regions.
“This use-dependent increase in local blood flow (functional hyperemia), mediated by mechanisms collectively termed neurovascular coupling (NVC), is essential for normal brain function and represents the physiological basis for functional magnetic resonance imaging,” said Nelson.
“Furthermore, deficits in cerebral blood flow (CBF) including functional hyperemia are an early feature of small vessel diseases (SVDs) of the brain and Alzheimer’s long before overt clinical symptoms.”
Cerebral blood delivery depends on mechanisms such as electrical signaling, which propagates through capillary networks to upstream arterioles to deliver blood, and calcium signaling, which fine-tunes local blood flow. For years, these mechanisms were thought to operate independently.
However, Nelson’s research reveals that these systems are deeply interconnected through E-Ca coupling, where electrical signals enhance calcium entry into cells, amplifying localized signals and extending their influence to neighboring cells.
The study demonstrated that electrical hyperpolarization in capillary cells spreads rapidly through activation of capillary endothelial Kir2.1 channels, specialized proteins in the cell membrane that detect changes in potassium levels and amplify electrical signals by passing them from cell to cell.
This creates a wave-like electrical signal that travels across the capillary network. At the same time, calcium signals, initiated by IP3 receptors—proteins located in the membranes of intracellular storage sites—release stored calcium in response to specific chemical signals.
This local release of calcium fine-tunes blood flow by triggering vascular responses. E-Ca coupling bridges these two processes, with the electrical waves generated by Kir2.1 channels enhancing calcium activity, creating a synchronized system that adjusts blood flow both locally and across wider distances.
Using advanced imaging and computer models, the researchers were able to observe this mechanism in action. They found that electrical signals in capillary cells boosted calcium activity by 76%, significantly increasing its ability to influence blood flow.
When the team mimicked brain activity by stimulating these cells, calcium signals increased by 35%, showing how these signals travel through the capillary network.
Interestingly, they discovered that the signals spread evenly throughout the capillary bed, ensuring that blood flow is balanced across all areas, without favoring one direction or another.
“Recently, the UVM team also demonstrated that deficits in cerebral blood flow in small vessel disease of the brain and Alzheimer’s could be corrected by an essential co-factor of electrical signaling,” noted Nelson.
“The current work indicates that calcium signaling could also be restored. The ‘Holy Grail,’ so to speak, is whether early restoration of cerebral blood flow in brain blood vessel disease slows cognitive decline.”
This discovery underscores the vital role of capillaries in managing blood flow within the brain.
By identifying how electrical and calcium signals work together through electro-calcium coupling, the research sheds light on the brain’s ability to efficiently direct blood to areas with the greatest demand for oxygen and nutrients.
This is especially significant because disruptions in blood flow are a hallmark of many neurological conditions, such as stroke, dementia, and Alzheimer’s disease.
Understanding the mechanics of E-Ca coupling offers a new framework for exploring treatments for these conditions, potentially leading to therapies that restore or enhance blood flow and protect brain health.
This breakthrough also provides a deeper understanding of how the brain maintains its energy balance, which is critical for sustaining cognitive and physical function.
Funding: The research discussed in this publication was supported by the National Institute on Aging (NIA) and the National Institute of Neurological Disorders and Stroke (NINDS) under grants K99-AG-075175 (A.M.), R01-NS-110656 (M.T.N.), RF1-NS-128963-01 (M.T.N.), and R01-NS-119971 (N.M.T.).
Additional funding was provided by the National Institute of General Medical Sciences (NIGMS) through grant P20-GM-135007 (M.T.N. & Mary Cushman), the National Heart, Lung, and Blood Institute (NHLBI) through grant R35-HL-140027 (M.T.N.), and the American Heart Association through a Career Development Award (856791 to A.M.) and a postdoctoral fellowship (20POST35210155 to A.M.).
Support was also received from the Totman Medical Research Trust (M.T.N.), the European Union Horizon 2020 Research and Innovation Programme (Grant Agreement 666881, SVDs@target, M.T.N.), and the Leducq Foundation Transatlantic Network of Excellence (International Network of Excellence on Brain Endothelium: A Nexus for Cerebral Small Vessel Disease, M.T.N.).
About this neuroscience research news
Author: Angela Ferrante
Source: University of Vermont
Contact: Angela Ferrante – University of Vermont
Image: The image is credited to Neuroscience News
Original Research: The findings will appear in PNAS